#Regulating the absorption spectrum of polydopamine
“#Regulating the absorption spectrum of polydopamine”
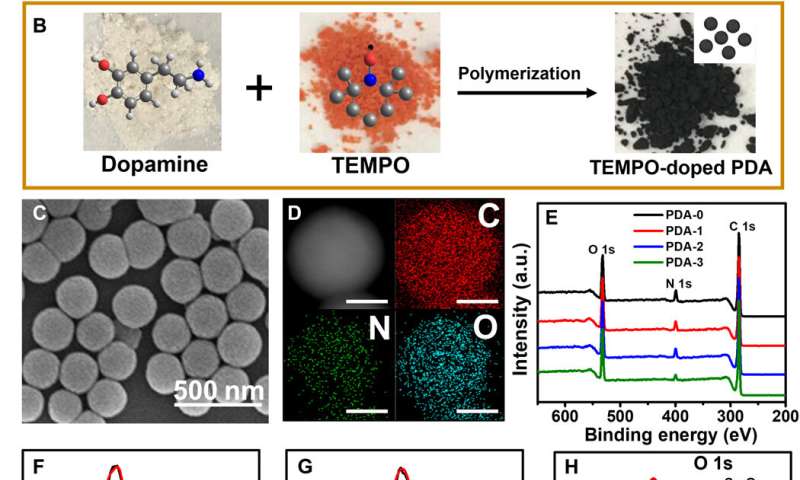
Polydopamine (PDA) is an advanced functional material and its emergent light absorption properties make it crucial for applications in materials science. However, it is challenging to rationally design and regulate PDA absorption properties due to its complex architecture. In a new report, Yuan Zou and a team of researchers in polymer science, optoelectronic materials and physical chemistry in China proposed a simple method to regulate the light absorption behaviors of PDA. To accomplish this, they constructed donor-acceptor pairs in the microstructures via connections between specific chemical moieties. They then used detailed structural and spectral analysis as well as density functional theory (DFT) simulations to confirm the existence of such donor-acceptor molecular pairs. The molecular pairs could decrease the energy bandgap (or energy gap where no electrons exist) and increase electron delocalization to enhance light absorption across a broad spectrum. The rational design of PDA nanoparticles with tunable absorption properties allowed an improved photothermal effect, which the team demonstrated with excellent performances during solar desalination. The work is now published on Science Advances.
Polydopamine
Inspired by the bio-macromolecular pigments of melanin, polydopamine (PDA) has received increasing attention for applications in surface engineering, photothermal therapy and bioimaging. The strong adhesive and light absorption properties of PDA can also benefit interfacial engineering during water remediation. Scientists have proposed many synthetic methods to prepare PDA nanomaterials, although with limited attention to regulate its absorption spectrum. The dopamine polymerization process is composed of several complicated pathways and therefore not fully understood. As a result, Zou et al. assumed that the construction of highly conjugated structures relative to donor-acceptor pairs in the PDA nanostructures could regulate the absorption spectrum of the sample. To accomplish that in this work, they developed a one-pot synthesis strategy to synthesize PDA nanoparticles (NPs) with tunable light absorption properties.
Synthesis and characterization
During the synthetic process, they conducted direct copolymerization of 2,2,6,6-Tetramethylpiperidine-1-oxyl (TEMPO) – a typical nitroxyl radical, onto dopamine in an aqueous solution. They doped the TEMPO moiety to the polydopamine microstructure by covalently connecting the molecule with 5,6-dihydroxyindole (DHI) and Indole-5,6-quinone (IQ) oligomers to narrow the energy band gaps of the material and improve light absorption behaviors of conventional polydopamine nanoparticles (PDA NPs). The scientists confirmed the outcomes by using electrochemical analysis, density functional theory simulations and spectral measurements. The work demonstrated an outstanding photothermal efficiency for the product that can be used in interfacial solar steam generation and seawater desalination.
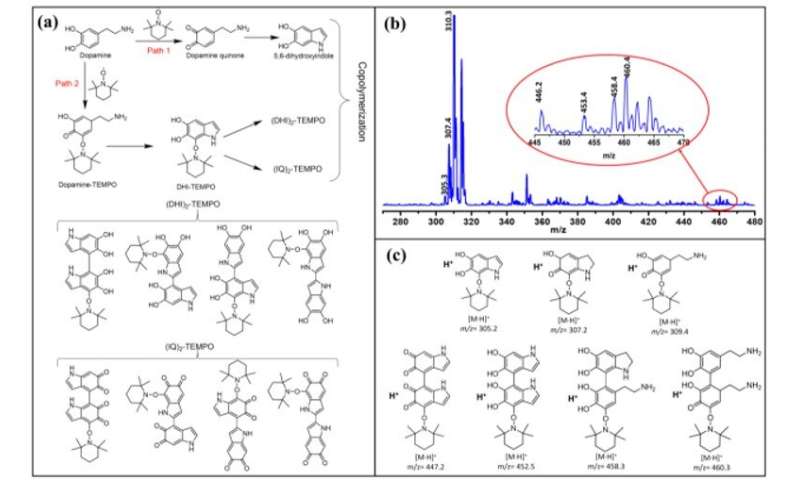
The scientists developed three types of PDA NPs (classified between 1 to 3) with different doping contents and similar particle sizes by tuning the initial concentration of TEMPO. They synthesized conventional PDA NPs through self-polymerization of dopamine in the presence of ammonium using a well-established method. They observed the resulting PDA sample characteristics using scanning electron microscopy, dynamic light scattering and Fourier transform infrared spectra (FTIR). Using X-ray photoelectron spectroscopy (XPS), they confirmed the existence of carbon, nitrogen, and oxygen elements in all PDA samples highlighting the successful preparation of TEMPO-doped PDA NPs. Based on the results, Zou et al. hypothesized two possible pathways to form the cross-linked macromolecular structure.
Enhanced light absorption and photothermal behavior of TEMPO-doped PDAs.
The team examined the light absorption capacity and total photothermal effect of these TEMPO-doped PDA NPs, where the product strongly absorbed light by catching and converting solar energy to heat energy efficiently with wide-ranging applications. During further tests, they dispersed PDA-3 in water at several concentrations for irradiation under lasers. When compared to many other outstanding photothermal materials, the TEMPO-doped PDA NPs showed better photothermal behaviors. For instance, Zou et al. noted how gold nanoparticles could suffer substantial loss of light absorbance after long-term irradiation due to structural destruction via the accompanying heat of the experimental conditions. The team contrastingly demonstrated how TEMPO-doped PDA NPs maintained enhanced light absorption capabilities with improved photothermal behaviors compared to conventional photothermal nanomaterials. The resulting material can serve as new generation photothermal agents to complete a variety of applications.
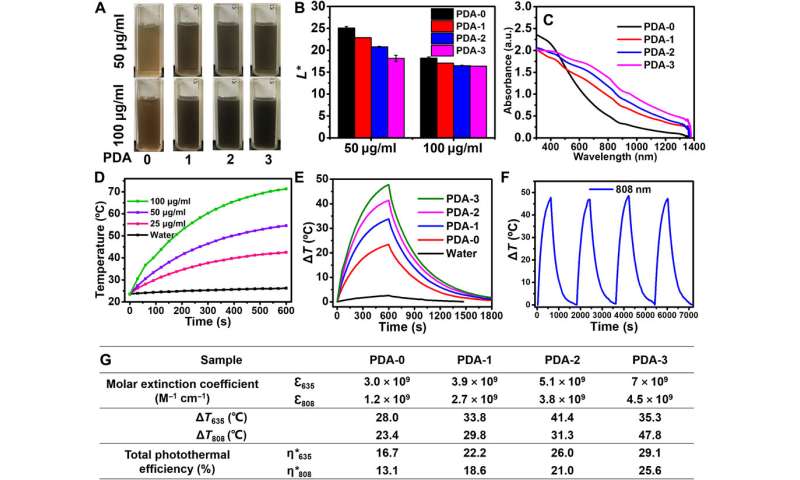
Analyzing the structure and absorption property regulation of TEMPO-doped PDA
The scientists noted the spontaneous formation of donor-acceptor microstructures in the TEMPO-based PDA system due to chemical conjugation between TEMPO and DHI, IQ and their oligomers during the polymerization process. This reaction contributed to the lower energy bandgap and enhanced light absorption of the product. To verify this, they calculated the optical bandgap value of different PDA samples in their aqueous solution forms alongside their electrochemical cyclic voltammetry (CV) to investigate energy bandgaps of all samples. They established the highest occupied molecular orbital (HOMO) and lowest unoccupied molecular orbital (LUMO) using the CV measurements and established the TEMPO unit as a donor fragment. As the doping concentration of TEMPO increased, the IQ moiety proportion also gradually increased, resulting in better delocalization of electrons for improved light absorption. The team hypothesized an increase in free radicals during PDA synthesis via TEMPO doping, which they tested and verified using electron paramagnetic resonance (EPR) measurements. Since the exciton-induced absorption (EIA) spectra did not rely on the amount of TEMPO doped to form the compound, the team credited it broadly to the presence of excitons in its additional constituents (such as DHI, IQ).
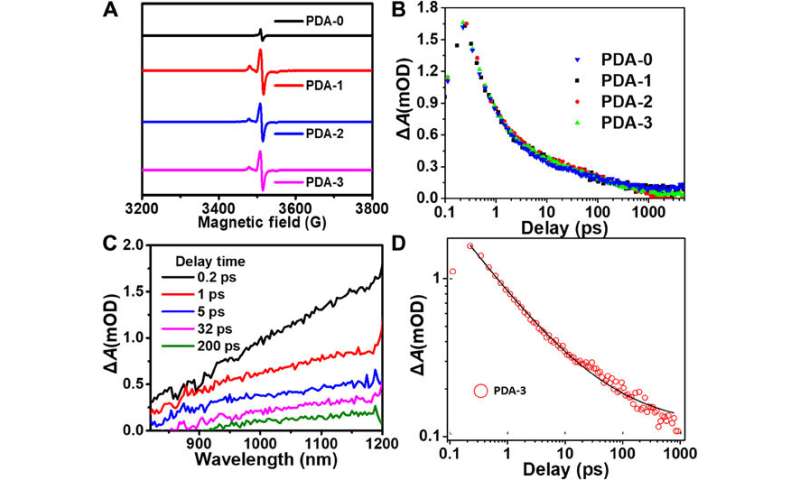
Applications of water desalination
The excellent photothermal and light absorption properties of TEMPO-based PDA made the material well-suited for applications in water steam generation and seawater desalination. Of the variety of samples, Zu et al. selected PDA-3 as the most promising candidate to develop the evaporation device. To accomplish this, they deposited the PDA-3 aqueous solution onto a cellulose membrane as a hydrophilic light absorber and prevented direct contact with water by using a thermal insulation layer such as polystyrene. When Zou et al. exposed the experimental setup to solar irradiation, they purified water by collecting condensed water from the solar steam. The PDA-3-coated cellulose membrane showed improved light absorption compared to control samples. The construct absorbed a majority of solar energy in the UV and visible regions. To understand solar vapor generation and photothermal evaporation performance, they measured the weight loss of water during evaporation and considered the energy conversion efficiency as an important index. The results indicated the feasibility of the device for desalination alongside efficient and durable activity.
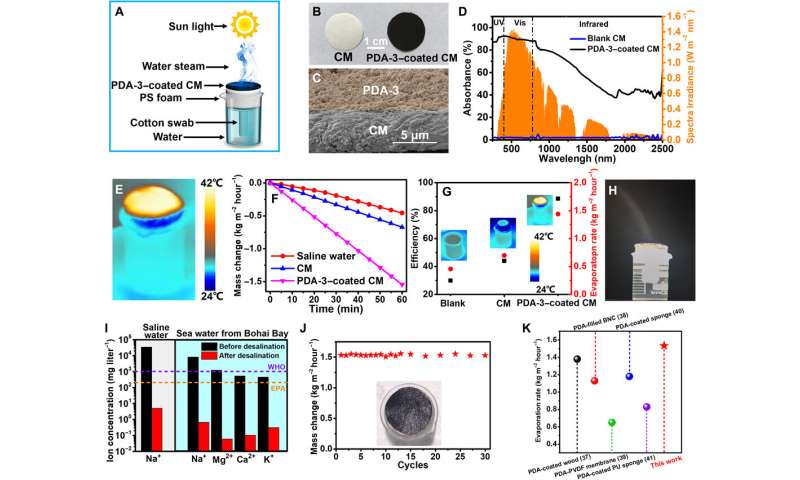
In this way, Yuan Zou and colleagues proposed a simple method to regulate the absorption spectrum of polydopamine (PDA) in a one-pot polymerization process in the presence of dopamine and TEMPO. The resulting nanoparticles had improved light absorption ability and photothermal effects when compared to conventional PDA nanomaterials due to the donor-acceptor structures in the PDA system. When they coated the resulting TEMPO-based PDA on the cellulose film, the construct acted as a sunlight absorber suited for water evaporation with a high efficiency of solar conversion and excellent rate of evaporation. The work will offer new opportunities for structural and functional PDA nanomaterials suited for light-harvesting applications.
More information:
Yuan Zou et al. Regulating the absorption spectrum of polydopamine, Science Advances (2020). DOI: 10.1126/sciadv.abb4696
Kaifeng Wu et al. Plasmon-Induced Hot Electron Transfer from the Au Tip to CdS Rod in CdS-Au Nanoheterostructures, Nano Letters (2013). DOI: 10.1021/nl402730m
S. Premi et al. Chemiexcitation of melanin derivatives induces DNA photoproducts long after UV exposure, Science (2015). DOI: 10.1126/science.1256022
© 2020 Science X Network
Regulating the absorption spectrum of polydopamine (2020, September 11)
retrieved 11 September 2020
from https://phys.org/news/2020-09-absorption-spectrum-polydopamine.html
This document is subject to copyright. Apart from any fair dealing for the purpose of private study or research, no
part may be reproduced without the written permission. The content is provided for information purposes only.
If you want to read more Like this articles, you can visit our Science category.
if you want to watch Movies or Tv Shows go to Dizi.BuradaBiliyorum.Com for forums sites go to Forum.BuradaBiliyorum.Com