#Metasurface enabled quantum edge detection
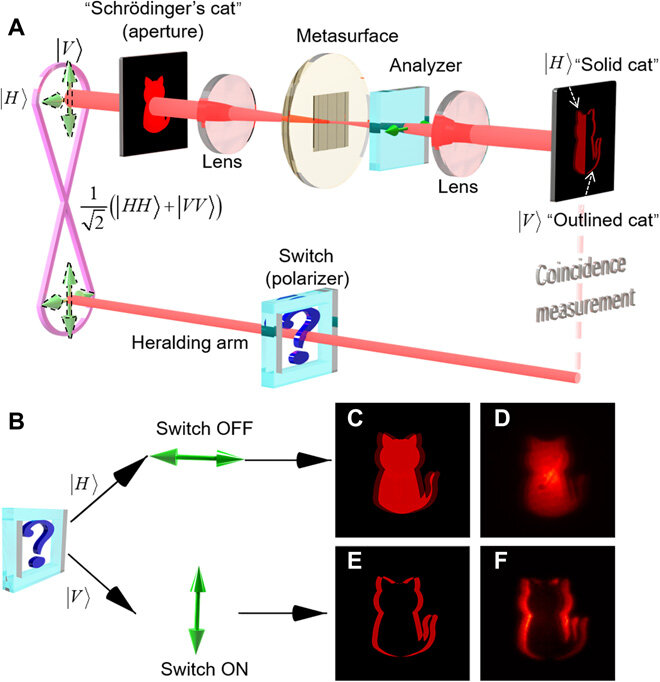
“#Metasurface enabled quantum edge detection”
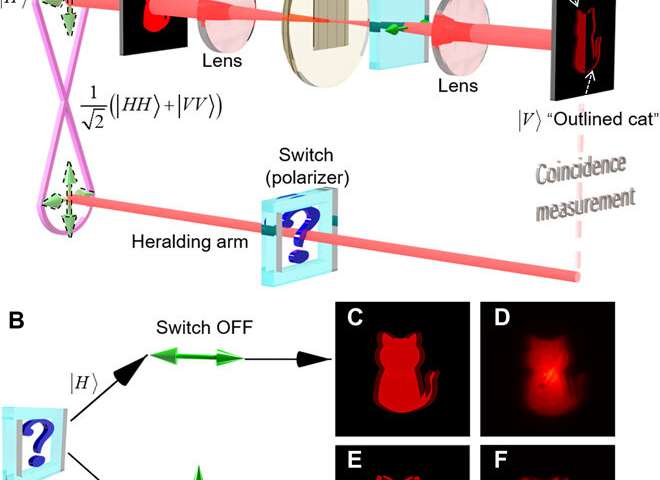
Metasurfaces provide unique platforms to realize exotic phenomena including negative refraction, achromatic focusing, and electromagnetic cloaking due to the engineered dielectric or metallic architectures. The intersection of metasurfaces and quantum optics can lead to significant opportunities that remain to be explored. In a new report now published on Science Advances, Junxiao Zhou, Shikai Liu and a research team in quantum information, nano-optoelectronic devices and computer engineering in China and the U.S. proposed and demonstrated a polarization-entangled photon source. They used the source to switch the optical edge mode in an imaging system to ON or OFF states based on a highly dielectric metasurface. The experiment enriched the fields of quantum optics and metamaterials as a promising direction toward quantum edge detection and image processing with a remarkable signal-to-noise ratio.
Combining quantum entanglement and edge detection
Photonic metasurfaces are two-dimensional (2-D) ultrathin arrays of engineered metallic or dielectric structures that can facilitate electromagnetic field manipulation of the local phase, amplitude and polarization. Researchers generally develop such capabilities for a variety of applications in classical optics. Quantum entanglement is essential in quantum optics for many applications including quantum cryptography, teleportation, superresolving metrology and quantum imaging. Recent efforts show a trend to combine the metasurface with entangled photons for potential applications in quantum optics. Edge detection is another factor that contributes to image processing to define the boundaries between regions in an image. It is a basic tool in computer vision to pre-process automations in medical imaging and forms a critical component of autonomous vehicles. Metasurface-enabled edge detection can be used in quantum optics to offer possibilities of remote-controlled image processing and cryptography. In this work, Zhou et al. therefore realized a polarization-entangled photon source and high-efficiency metasurface enabled switchable optical edge detection method. The combined strategy showed a high signal-to-noise (SNR) ratio at the same photon flux level (the number of photons per second per unit area).
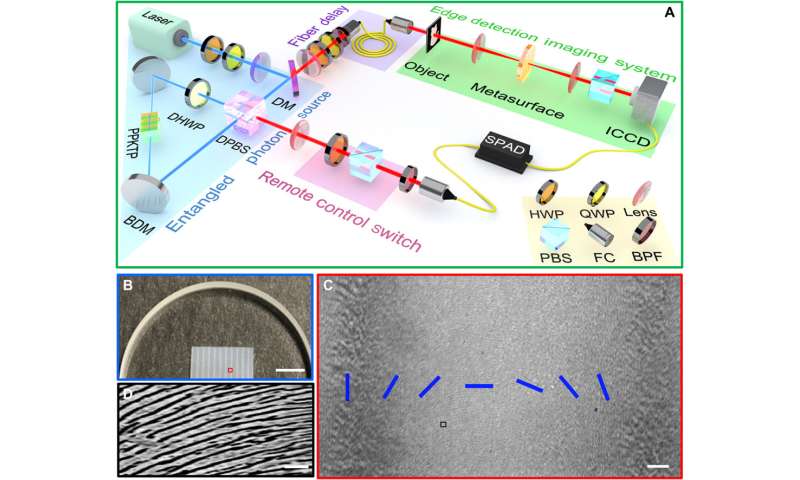
Using the “Schrödinger’s cat” concept
Zhou et al. used the Schrödinger’s cat concept to illustrate the expected performance of the switchable quantum edge detection scheme. They reviewed the basic principle of edge detection based on classical continuous wave (CW) light-illumination. In the experimental setup, the edge detection imaging arm was independent of the entangled source and the heralding arm, as well as the coincidence measurement components. When the incident photons achieved a horizontal polarization state, the beam of illuminated light passed through a cat-shaped aperture and an engineered metasurface to separate into a left- and right-handed overlapped polarized image with a horizontal shift. The overlapped components then passed through a horizontally oriented analyzer to form a ‘solid cat’ image. If, however, the incident photons were vertically polarized, the overlapped components recombined to a linear polarized component that is completely blocked by the analyzer to only form an outline of a cat. The researchers therefore used polarization-entangled photons as a source of illumination to develop quantum switchable edge detection in this way.
The experimental setup and polarization-entangled photon pairs
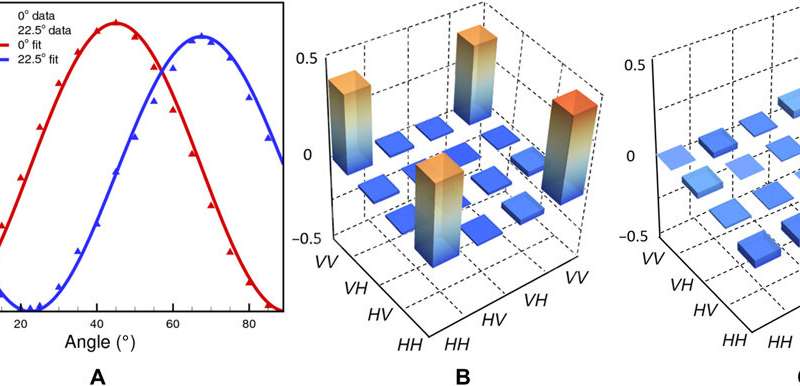
The researchers generated polarization entangled photons using a spontaneous parametric down-conversion process in a 20-mm-long type II phase-matched periodically poled potassium titanyl phosphate (KTiOPO4/PPKTP) crystal embedded in a Sagnac interferometer. They set the temperature of the crystal to 17 degrees Celsius and used two broadband dielectric mirrors and a dual-wavelength polarization beam splitter to form the self-stable Sagnac interferometer. They then used a continuous wave single-frequency diode laser at 405 nm to generate the pump beam focused by a pair of lenses with optimized focal lengths to attain a beam waist approximating 40 microns at the center of the crystal. To balance the power in the clockwise and counter-clockwise-directions, Zhou et al. used a quarter-wave plate (QWP) and a half-wave plate (HWP) in front to the Sagnac loop.
Using a dual-wavelength polarization beam splitter, they separated the down-converted photon pairs pumped by two counter-propagating beams, to send one into the imaging arm and the other to heralding arms, respectively. Zhou et al. also designed the metasurface employed in the setup using the Pancharatnam-Berry phase and fabricated it by scanning a femtosecond pulse laser within a silica slab. Then using scanning electron microscopy, they observed self-assembled nanostructures in the silica slab and showed their origin under intense laser irradiation to generate the metasurface. The team briefly described the quantum state preparation for the polarization entangled degenerate photon pairs generated from the Signac loop. They used the Bell state (the simplest example of nonseparable quantum entanglement) for this work by adjusting the experimental setup. Zhou et al. quantified the entanglement quality of the two-photon state using quantum tomography and reconstructed two-photon density matrix measurements.
-
The switchable edge detection demonstration. (A to D) The metasurface sample orientation, which is aligned with the xy plane. The inset yellow arrows indicate the phase gradient direction of the metasurface. (E to H) The images of the whole object comprising the separated LCP and RCP components, which is the OFF state of the edge detection mode. (I to L) The images reveal edges along different directions, which is the ON state of the edge detection mode. Photo credit: Junxiao Zhou, University of California, San Diego. Credit: Science Advances, doi:10.1126/sciadv.abc4385
-
Entanglement-enabled quantum edge detection has high SNR. (A and C) The edge detection images are triggered by the heralding detector. (B and D) Direct images where the ICCD is internally triggered. (C) and (D) are taken along the white dashed lines in (A) and (B), respectively. Credit: Science Advances, doi:10.1126/sciadv.abc4385
Quantum-entanglement enabled quantum edge detection
After confirming the quality of generated polarization-entangled photon pairs, they demonstrated switchable quantum edge detection. To accomplish this, they prepared the photons in horizontal or vertical linear polarizations states using the setup and coupled the photons into the fiber and sent them to the edge detection image system to capture the final alternative image via an intensified charge-coupled device camera (ICCD). For instance, Zhou et al. obtained two overlapped images with a tiny shift, where the shift direction aligned with the phase gradient direction of the metasurface. When they increased the period of the metasurface structure, they decreased the shift between the two overlapped images to achieve high-resolution edge detection. The quantum edge detection scheme had another advantage due to its high signal-to-noise (SNR) ratio, where the team could significantly reduce the ambient noise in the setup, where noise only accumulated in a very short timeframe. By contrast, in classical optics, the noise would continue to accumulate. As proof of concept, they acquired an edge image with remarkable SNR for improved entanglement-enabled experimental quantum edge detection.
Outlook
In this way, Junxiao Zhou, Shikai Liu and colleagues combined quantum entanglement-enabled quantum edge detection using a metasurface filter combined with a polarization-entangled source. The metasurfaces provided ultrathin and lightweight optical elements with precisely engineered phase profiles to obtain a variety of functions to form a more compact and integrated system. The setup will assist the conception of security applications including image encryption and steganography. The method also offers an appealing signal-to-noise (SNR) ratio suited for a variety of photon-hungry imaging and sensing applications in biomedicine, including tracking enzymatic reactions and observing living organisms or photosensitive cells.
Researchers demonstrate nondestructive mid-infrared imaging using entangled photons
Zhou J., Liu S. et al. Metasurface enabled quantum edge detection, Science Advances, DOI: 10.1126/sciadv.abc4385
Yu N. Capasso F. et al. Flat optics with designer metasurfaces, Nature Materials, doi.org/10.1038/nmat3839
Bouwmeester D. et al. Experimental quantum teleportation, Nature, doi.org/10.1038/37539
© 2020 Science X Network
Citation:
Metasurface enabled quantum edge detection (2020, December 29)
retrieved 29 December 2020
from https://phys.org/news/2020-12-metasurface-enabled-quantum-edge.html
This document is subject to copyright. Apart from any fair dealing for the purpose of private study or research, no
part may be reproduced without the written permission. The content is provided for information purposes only.
If you liked the article, do not forget to share it with your friends. Follow us on Google News too, click on the star and choose us from your favorites.
For forums sites go to Forum.BuradaBiliyorum.Com
If you want to read more Like this articles, you can visit our Science category.